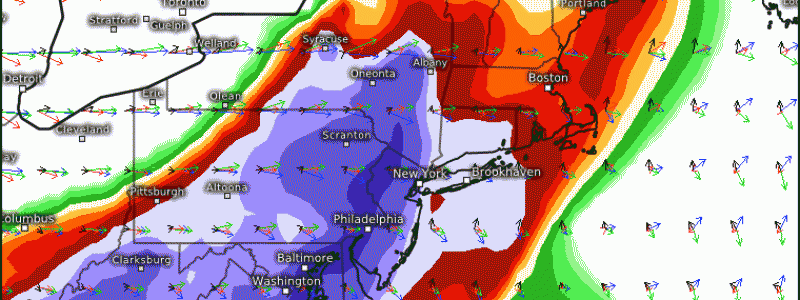
The Springfield Tornado, Ten Years Later: A Retrospective Analysis
Every May, there are a few days that the Plains of the central US see an astonishing feat of nature. As sinking troughs incite low-level mass removal, brisk southerly flow pulls north Gulf moisture from the south and, a little higher up, hot and dry air from the Mexican plateaus. Where these overlapped airmasses are encroached from above by brisk midlevel flow, erupting updrafts can readily organize and drop significant tornadoes. If it weren’t so devastating, the machine-like ability of the central North American geography to frequently output tornadoes with the minimal addition of a trough would be miraculous.
But take the ingredients just slightly out of balance, and it becomes significantly harder to generate intense tornadoes. This is the case in the northeastern US, where a number of tweaks on the formula mastered by the Plains conspire to substantially reduce the odds of tornadic violence. Far from the extratropical system reset button provided by the Rockies, lows are typically more mature, with more of a separation between southerly flow and the midlevel jet stream. Additionally, a lesser proximity to both sources of atmospheric instability, the Gulf of Mexico and the adjacent Mexican plateau, means convective overturning upstream generally degrades the quality of the airmass before it can advect all the way to the northeast. And, at a higher latitude, a relatively meager sun angle additionally inhibits the air from achieving the jet-fuel instability common to the southwest.
This doesn’t mean tornadoes don’t occur, though. The northeast, from Pennsylvania to Maine, averages dozens of tornadoes a year. Most are weak, but a significant tornado or two a year in this expansive region is generally a given if mesoscale conditions are most favorable, often in small zones of enhanced kinematic support come July and August. And sometimes, perhaps once a decade, a true atmospheric anomaly can occur: a Plains-esque environment manages to develop in the northeast.
When it manages to happen, it’s really nothing short of a perfect storm, temporally and spatially. It takes a flow regime supportive of carrying an EML thousands of miles from the depths of the southwest without convective degradation, and southerly flow capable of snaking moisture from the Gulf, a multi-day affair. It takes a cyclone oriented just the right way to midlevel support such that flow aloft can overspread the unusually explosive warm sector with favorable shear, and also requires a strong enough storm to pull north a low level jet strong enough for tornadogenesis. These factors and their unlikely overlap are how, ten years ago today, a high-end EF3 roared through more than 35 miles of southern Massachusetts, bringing a corridor of death and destruction almost unheard of for the state.
It started, as these things often do, with innocuous anticyclonic flow over the eastern half of the US.
While bowling zones of high pressure aren’t typically thought of as precursors for significant severe weather, remember that the northeast is a finicky place to get tornadoes. A requirement for blockbuster environments is the elevated mixed layer, sourced from the Mexican Plateau. The airmass massively increases lapse rates, boosting instability, but is easily degraded by convection, which means it can’t usually make it all the way to the northeast unless advecting flow is unsupportive of widespread thunderstorm activity. And what’s more unsupportive than a high pressure dome!
The long-lasting predecessor anticyclone allowed a strong EML, ID’d by high lapse rates from 500 to 700mb, to pool over the eastern US by the end of May.
This antecedent airmass set the stage for a ‘perfect storm’ of destabilization when, early on the 31st, a trough pivoting from the Plains towards the Great Lakes began to inject deep moisture into the top of the eastern US anticyclone. This meant that, though flow in the low levels was westerly or even northwesterly in the northeast, moistening was able to begin in earnest west of the Hudson.
By the evening of May 31st, the confluence of deep moisture and a strong EML meant an axis of unusually extreme instability was present into Pennsylvania and New York. Forcing for ascent and organizing shear were still separated to the northwest, so severe weather was isolated despite high-end thermodynamics.
While kinematics may have been missing from the equation over the northeast on the 31st, they were not absent from North America by any means. In fact, a large and deep midlevel low was centered over Canada. Exit region flow exceeded 80 knots, and attendant mass removal supported a strong but well-occluded cyclone with a warm sector ‘fed’ by a strengthening southerly low level jet. But the cyclone was too far northwest to provide the type of widespread lift, organizing shear, and spin-inducing helicity required for a tornado outbreak amidst the rocking instability present on 5/31. This equation would change when, early on the 1st, a pivoting internal shortwave forced the midlevel closed low and attendant troughing to dive ESE through the Great Lakes.
As the exit region of the midlevel trough surged towards New England on June 1st, 2011, it was accompanied by a well timed late-stage cyclone occlusion process that forced secondary development along the horizontally oriented boundary just north of the Canadian border. As low level flow responded by fanning out broadly between the original and secondary cyclones, there was a sudden surge of kinematic support throughout the atmosphere that overspread the incredible instability at the surface. Suddenly, as daylight heating peaked over the eastern half of the northeast ahead of a surging attendant cold front, the perfect storm was happening.
It wasn’t long before updrafts, forced vertically by the cold front and lee troughing to the east, rapidly exploded upwards amidst high-end CAPE and were organized into long-lived severe storm cells by brisk midlevel flow. As surging, veering low level flow began to rotate these updrafts, the storms strengthened into intense discrete supercells across the northeast.
One cluster of storms entering western Massachusetts benefitted from a coincidental combination of storm-scale mergers and an incredibly favorably mesoscale parameter space. A broad mesocyclone amidst the most aggressive supercell began to dramatically tighten and strengthen as the storm took on a well defined hook echo on approach to Springfield. East of the city, a tornado rapidly developed, quickly strengthening to a powerful EF3 tornado around 0.5 miles wide that tracked just south of the city center.
As the parent supercell began to settle into a favorable equilibrium within a very favorable environment, the couplet continued to intensify while the reflectivity took on a pronounced hook echo. As the tornado roared through suburb after suburb, a massive debris ball would also develop. The result was a textbook high-end radar presentation as the storm moved through Monson and north of Southbridge.
The tornado would end up tracking for around 38 miles, causing catastrophic damage to neighborhoods and completely deforesting wooded areas along a half mile wide path. It was officially delineated as a high end EF3, and some consider that to be an under-rating. The tornado killed three and injured hundreds, a significant human toll that could have well been worse in the highly populated and often ill prepared northeast had it not been for warnings with a significant lead time.
As far as I’m aware, there hasn’t been a tornado at or above EF3 intensity since that day in 2011. Of course, it’s only a matter of time before another ‘perfect storm’ happens, and a few environments have been within spitting distance (9/8/2012, 5/15/2018, 10/2/2018, or 5/15/2020, for example). But regardless of what the future may bring, Springfield will long be remembered by meteorologists in the northeast for its intensity, human toll, and environment.